#115 Fusion Q-Values and Breakeven Explained
Return to ITER Power Facts Main Page
By Steven B. Krivit
April 8, 2022
Explained in this article:
— The difference between Qscientific and Qengineering
— The difference between reaction gain and reactor gain
— The difference between scientific breakeven and engineering breakeven
— The difference between Fusion Power and Fusion Power
— The fusion term “scientifically feasible”
— The relevance of experimental fusion results to possible commercial fusion prospects
James McKenzie was the vice president for business at the U.K. Institute of Physics from 2016 to 2020. In March 2022, McKenzie wrote an article in Physics World enthusiastically promoting public support of fusion. He explained why:
Once you achieve fusion, you need to generate more energy than you put in, so that the ratio Q > 1. But a breakeven result has so far never been achieved by a fusion reactor here on Earth. In fact, what you really want is a Q between 5 and 10 so that your reactor produces a useful amount of power.
Full stop. A reactor, like ITER or SPARC, designed to reach a “Q” of 10, cannot produce a useful amount of power.
Fusion experts have had a long history of creating misunderstandings among people who are not experts in the field. McKenzie was only the latest casualty. Even Daniel Clery, Science magazine reporter and author of a popular book on fusion, wrote just a few days earlier that “ITER is designed to show net energy output can be achieved.”
In this explainer, I will clarify the various meanings of “Q” and the proposed values associated with achieving net energy in fusion reactors.
Reaction Performance Q vs. Reactor Performance Q
Fusion researchers use a shorthand notation, based on the capital letter Q, to describe fusion reaction performance. They also use the capital letter Q to describe fusion reactor performance. Reaction performance applies to only the physics reaction, the particles.
Alternatively, reactor performance applies to the overall reactor. Although the physics reaction provides useful information to physicists, only reactor performance can inform us, and answer the age-old question, “How close are we to obtaining useful power from fusion?”
A reasonable question is why fusion scientists use the Q denotation rather than describe reactor performance more simply, using the net power output produced by a fusion reactor. The answer is that, after 70 years and more than 100 experimental fusion reactors, no fusion reactor has produced potentially usable net power.
Perhaps more important, fusion scientists must design reactors first by developing a specification for projected reaction performance, and only then can they calculate projected reactor performance.
The use of capital Q to denote performance is not limited to fusion. Capital Q is a generic concept used in science to designate power or energy gain or loss. In physics, this shorthand can refer to fission, fusion, and nuclear decay reactions.
Fusion researchers have also developed two key milestones that are directly associated with Q-values: scientific breakeven and engineering breakeven. Discussing “breakeven” with a lay audience without clarifying which breakeven is a poor practice, but it is commonplace.
Qscientific
The first Q-value is called Qscientific. Q values followed by the subscript “scientific,” “fusion” or “DT” are usually synonymous. “DT” refers to the most common fuel, a mixture of deuterium and tritium.
Qscientific refers to the ratio of heat produced by a fusion reaction to heat consumed by the fusion reaction. Explained a little more technically, Qscientific is the ratio of the thermal power produced by a fusion plasma to the injected thermal power used to heat that plasma. Technically, heat is not measured directly in fusion reactors, but it is imputed based on the measured kinetic energy of the fusion-produced neutrons.
Qscientific is concerned with only the amplification of the heating power. It does not consider the electrical power required to produce that heating power. Nor does Qscientific consider the electrical power required to operate the rest of the reactor.
By convention, in fusion science, if Q is written alone, without any denotation, it implies Qscientific. Writing Q without any denotation to a lay audience is a poor practice, but it is also commonplace.
When the rate of heat produced by a fusion reaction equals the heat consumed by the fusion reaction, this is called “scientific breakeven.” Mathematically, this is a ratio of 1; that is, Qscientific = 1. Alternatively, for example, if the heat produced by a fusion reaction is 50 megawatts and the heat consumed by the fusion reaction is 100 megawatts, then we have a Qscientific value of 0.5.
When scientists use the phrase “scientifically feasible,” they mean the anticipated condition when a fusion reaction achieves scientific breakeven. But achieving “scientific feasibility” does not mean that the overall reactor has proven that fusion is feasible as a practical source of energy. A reactor that achieves nothing more than “scientific feasibility” would still consume more power than it produces. Therefore, the terms scientific breakeven, scientific feasibility, and Qscientific are useful only in the context of scientific research, rather than in a practical context.
A Qscientific value greater than 1, and its associated scientific breakeven milestone, is a necessary but insufficient condition for practical power generation. Two more steps are required.
Qengineering
Q followed by the subscript “engineering” refers to the ratio of the electrical power produced by a fusion reactor to the electrical power consumed by the fusion reactor. Any Qengineering value greater than 1 means that the reactor can produce net electrical power. If a particular reactor design is not configured to convert its thermal output to electric, the thermal output can be multiplied by 0.4 to get the equivalent electric output.
A scientific paper published by Jonathan Menard, the deputy director for research at the Princeton Plasma Physics Laboratory (PPPL), and his colleagues contains graphs that describe a variety of fusion reactor design variables. These variables, such as reactor size and neutron flux, are of interest to fusion scientists. However, for the rest of us, the scales alone, displayed in the vertical axes in graphs in the paper, provide a meaningful way to see and compare the relationship between the two types of Q-values. I’ve taken these scales, placed them side-by-side, and used them to show the relationship between Q-values in some tokamak reactor designs.
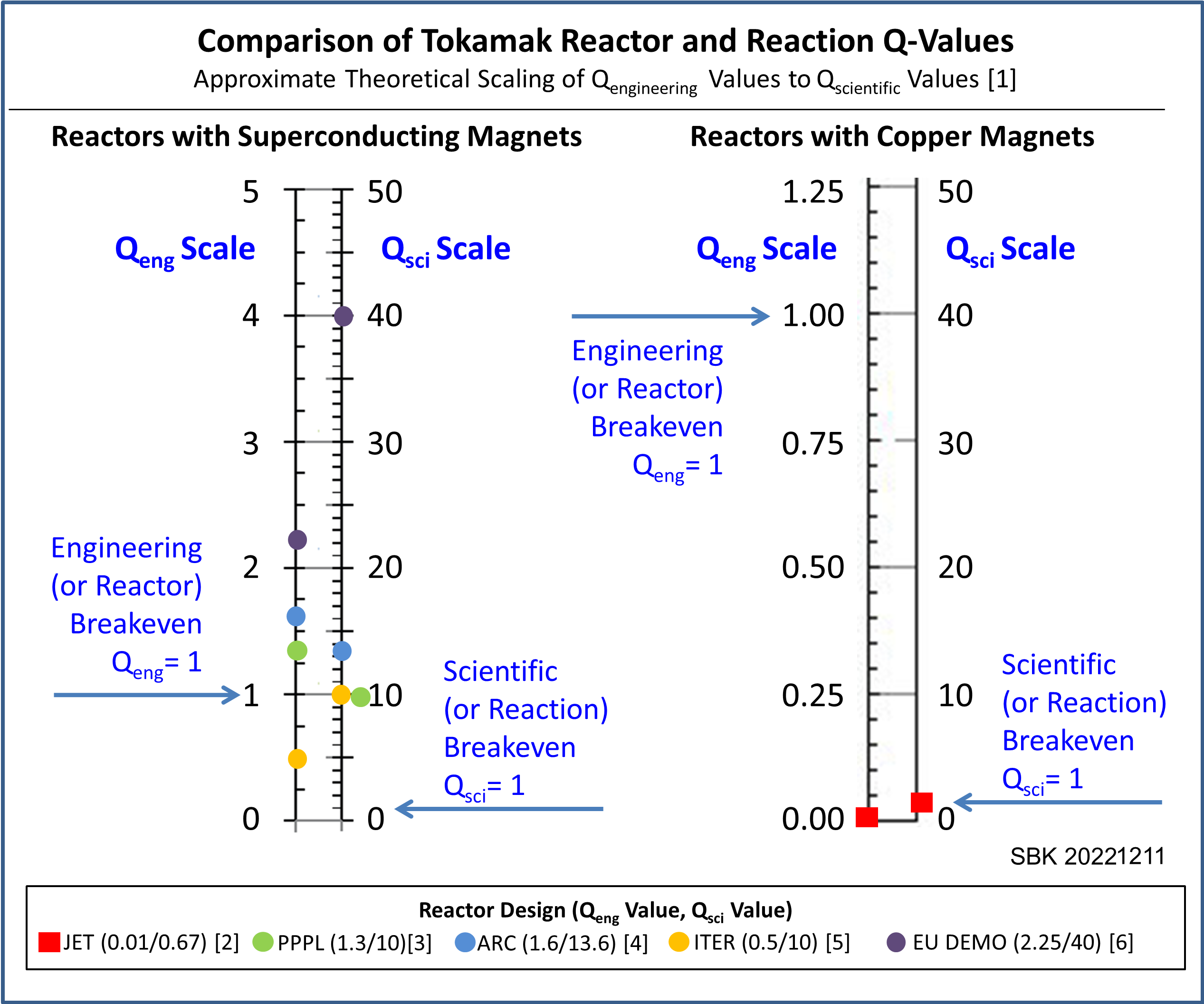
Graph updated April 4, 2022, to correct ARC Qeng value.
Copper Versus Superconducting Magnets
The most popular type of fusion reactor is called a tokamak. This category of reactors has two sub-categories: those that use the older, copper magnets and those that use the newer, superconducting magnets. Most fusion scientists expect that only the newer, superconducting reactor designs have the potential to produce useful electrical energy.
No experiments in fusion reactors with superconducting magnets and deuterium-tritium fuel have taken place yet. Thus, to see the state-of-the-art experimental results, we must look at the older copper-magnet reactor designs.
The most powerful result took place on Oct. 31, 1997, in the Joint European Torus (JET) reactor in the United Kingdom. In this experiment, the Qscientific value was 0.67, and the Qengineering value was 0.01. I show these values with the two red squares in the graph above.
No scientist, to my knowledge, has published the Qengineering value for JET — and for good reason. JET was never intended for reactor power gain, only for reaction power gain. I obtained the reactor input power value — 700 megawatts of electricity — on Dec. 1, 2014, from Nick Holloway, who was then the spokesman for the United Kingdom Atomic Energy Authority. With that, I calculated the Qengineering value: [(16 MW * 0.4) / 700 MW]. (The value of 40% is a reasonable assumption at which thermal energy can be converted into electricity based on current steam turbine technology.)
The scales show that, in order to make one net Watt of electricity, that is, achieving a Qengineering value greater than 1, a copper-magnet-based reactor would need to achieve a Qscientific value above 40. The consensus in the fusion community is that this is never likely (but not impossible) to happen with a copper-magnet-based reactor.
Most of the 700 megawatts that the JET reactor consumed was used for the magnets. Superconducting magnets are significantly more efficient.
The ITER design specification, which uses superconducting magnets, calls for a Qscientific value of 10. The design specification does not set a target for Qengineering. No scientist, to my knowledge, has published the Qengineering value for ITER. As with JET, ITER was never intended for net reactor power gain, only reaction power gain.
I obtained the minimum reactor input power value — 300 megawatts — in the summer of 2017 and I published it on Oct. 6, 2017. This was the first time it appeared in any news article or Web page, though similar numbers appeared in deeply buried technical documents. When I later located these documents, they indicated that the minimum reactor input power value was even higher, up to 440 megawatts of electricity. All of the references and sources are here.
Using the more conservative number of 300 megawatts, if ITER achieves its Qscientific goal of 10, the Qengineering value will be about 0.73. This means that the overall reactor, if it works correctly, will not produce any net power or net energy. I show these values with the two yellow dots in the graph below.
All things being equal, each colored pair on the graph would appear on the scales at the same height. However, every reactor design is based on unique variables that will determine each reactor’s specific Qscientific and Qengineering values.
As well, designers of reactors have the option to choose various design parameters that may be conservative or optimistic. The scales, therefore, provide a baseline for comparison. The values for JET, shown in red squares, are the only experimental results shown on the graph. All other values shown are design projections.
The green circles represent a publicly funded fusion reactor design by the Princeton Plasma Physics Laboratory. The blue circles represent a 2014 reactor design by students and professors then at the Massachusetts Institute of Technology. The purple circles represent a publicly funded fusion reactor design by the EUROfusion organization for the EU DEMO reactor. Menard confirmed the PPPL Q-values in an e-mail to me. Hartmut Zohm, the head of the tokamak scenario development division at the Max Planck Institute for Plasma Physics also confirmed the EU DEMO Q-values in an e-mail to me.
Most fusion scientists believe that, for a superconducting reactor, a Qscientific value greater than 25 would be the minimum needed to demonstrate a proof of concept for practical electricity production from fusion.
Triple-Product Values Do Not Show Power or Energy Gain
Fusion advocates tend to create a lot of news media attention for every incremental step they take forward. But “world-record” achievements of plasma temperature or field strength of magnets do not tell us anything about power gain because neither measures power production or power consumption.
Not even the much-trumpeted “triple-product” value — a scientific term that measures the combined values of plasma density and plasma confinement time and temperature — tells us about power or energy gain. That’s because the “triple-product” value, although it imputes power gain in a fusion reaction, doesn’t measure power production or power consumption in a fusion reactor.
That’s why the graph produced by Department of Energy ARPA-E program managers, Samuel Wurzel and Scott Hsu, is irrelevant to any discussion of power gain in fusion reactors. It is only relevant to fusion reactions and, at that, is only a theoretical inference. Nevertheless, the Wurzel-Hsu graph has recently been used by fusion promoters.
Andrew Holland is the founder of a company called Fusion Industry Association, which took over the fusion advocacy role played for many decades by Stephen Dean, through his company Fusion Power Associates. Holland displayed the Wurzel-Hsu graph in a presentation he gave to the President’s Council of Advisors on Science and Technology (PCAST) on Jan. 21, 2022.
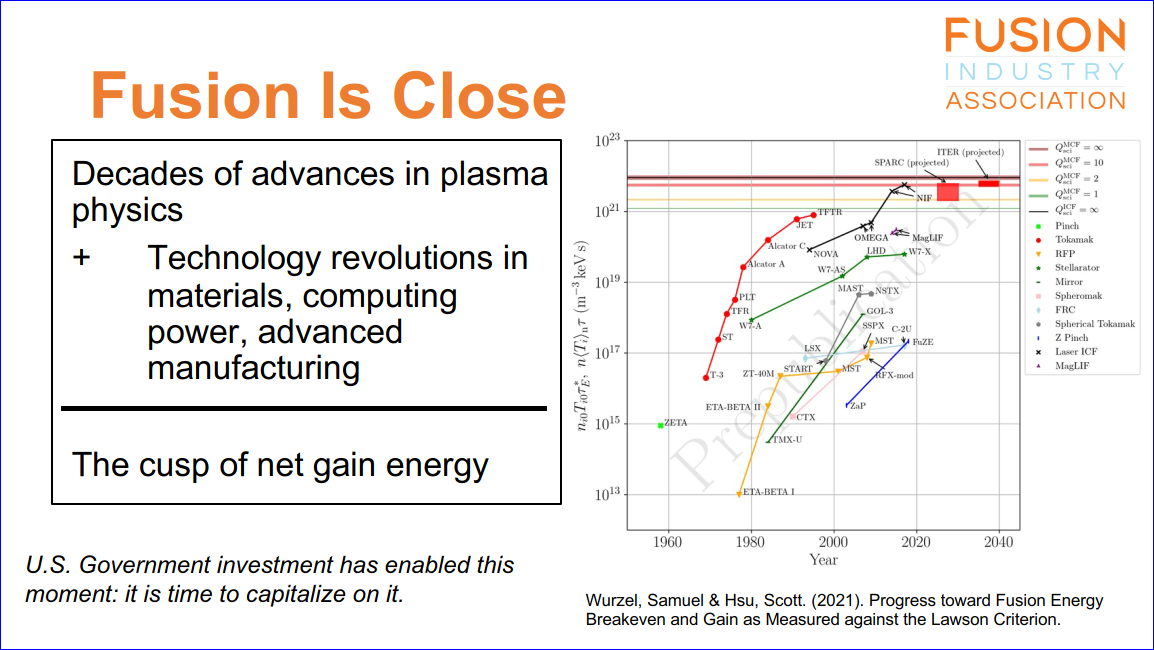
Slide displayed by Andrew Holland to the President’s Council of Advisors on Science and Technology on Jan. 21, 2022
None of the triple-product values or curves in the Wurzel-Hsu graph that Holland displayed to members of PCAST showed measurements of power or energy. This makes Holland’s statements that “fusion is close” and on “the cusp of net gain energy,” essentially meaningless.
The Wurzel-Hsu graph is not even relevant for progress in triple-product values. That’s because Wurzel and Hsu cherry-picked their values for tokamak reactors. They elected not to display the less-favorable triple-product values beyond 1995. They showed only the values up to 1995, displayed below in red. Had they continued to report the subsequent triple-product values, the graph would have included the values I added, shown below in blue. Full details on the story are here.
On March 17, 2022, U.S. Secretary of Energy Jennifer Granholm announced that Hsu had been appointed to the new position to coordinate fusion research at the Department of Energy.
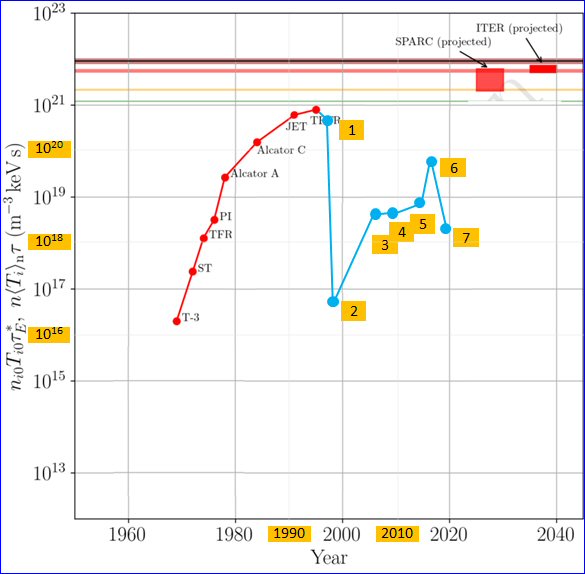
Wurzel-Hsu graph showing progress in triple-product values, with blue values added by Krivit
A few months earlier, on Oct. 19, 2021, Richard Hawryluk, the former interim laboratory director at the Princeton Plasma Physics Laboratory, also promoted fusion to the PCAST members. Here’s a slide he displayed to show fusion performance.
The graph on the left shows triple-product values. As I mentioned above, triple-product values do not and cannot measure reactor power or energy gain because they do not measure or account for reactor input power.
The more serious problem with the triple-product graph Hawryluk displayed is that, although it does show an increase in triple-product values, it shows that the highest values were obtained when the plasma durations were shortest: a tenth of a second. That’s not encouraging for a future source of energy. I explained more about this graph in slides 61 and 62 in “When Will We Get Energy From Nuclear Fusion?”
What About the New NIF Result?
The graph on the right of Hawryluk’s slide does, in fact, show energy measurements. They are the results from the National Ignition Facility’s laser fusion device. The graph certainly gives the appearance of dramatic progress in 2021, with a big pink line on the right showing fusion energy production of 1.35 megajoules.
Most of us never deal with Joules in our daily lives, let alone megajoules, so 1.35 megajoules might sound like a lot. It’s enough energy to boil one kettle of water. But to produce 1.35 megajoules of energy, the NIF device consumed 400 megajoules of energy, which Hawryluk did not explain. That means that NIF lost 99.6 percent of the energy it consumed. I explained more about the 2021 NIF result in this article and three follow-up articles beginning on Feb. 2, 2022.
What About the New JET Result?
The new 2021 result from the Joint European Torus (JET) fusion reactor, reported in February 2022, suffers from the same fusion hyperbole. JET produced 59 megajoules of energy last year. It did, in fact, double the energy that it produced 25 years ago. But both experiments consumed 3,500 MJ of energy. So in 1997, JET lost 99.4 percent of the energy it consumed. In 2021, JET lost only 98.3 percent. That is one-tenth of one percent improvement in 25 years.
Other Ambiguities
Press releases from fusion organizations, promotional literature, and quotes from fusion representatives often use the phrases “net positive energy” and “more power out than in.” Commonwealth Fusion Systems uses the phrase “net energy from fusion.” It pays to scrutinize such statements carefully. The vast majority of such phrases apply to only the reactions, not the reactors. People in the fusion business who use these phrases rarely explain this distinction, or if they do, they don’t do so transparently.
The Third Breakeven
If and when fusion scientists achieve scientific breakeven, and if and when they subsequently achieve engineering breakeven, a third milestone would be needed before fusion could become a practical source of energy. It’s called economic breakeven. This would occur when the cost of the produced energy equals the cost of the energy consumed plus the cost of the fuel.
Fifty years ago, fusion researchers began proposing reactor designs that they claimed would achieve engineering breakeven. In this context, the recent plethora of claims of near-term fusion reactors achieving not only the first and second levels of breakeven but also economic breakeven strains credulity. For this reason, I have not attempted to display economic breakeven in the set of scales in my Q-value comparison graph.
Coming back to McKenzie’s hope for a fusion reactor that produces a useful amount of power, the only relevant comment may be a comparison of economic breakeven to a nuclear fission power plant. A small electricity-producing fission reactor like the Ginna plant has a Qengineering value of 12. Let’s assume that the Ginna plant exceeds economic breakeven by a margin sufficient for its fission power to be sold profitably. Now let’s look for a Qengineering value of 12 for a fusion reactor in my graph. It would be off the scale. It would require a Qscientific value greater than 100. This is asking a lot for an industry that has never achieved a Qscientific value greater than 1.
Disclosure: Steven Krivit and New Energy Times do not have any business or financial affiliation with any energy research, energy company, or energy investment. This report is produced and distributed at no charge, as a public service, without sponsorship, advertising, or monetization.
References:
- Scales are based on graphs in J.E. Menard et al (2016) Nuclear Fusion 56 106023
- JET Q-values based on N. Holloway e-mail to S. Krivit, Dec. 1, 2014
- PPPL Q-values based on Menard, Jonathan E., Grierson, B.A., Brown, T., Rana , C., Zhai, Y., Poli, F.M., Maingi, R., Guttenfelder, W., and Snyder, P.B.,”Fusion Pilot Plant Performance And The Role Of A Sustained High Power Density Tokamak,” (2022) Nuclear Fusion 62 036026, and communication with J. Menard.
- ARC Q-values from B.N. Sorbom, J.Ball, T.R. Palmer, F.J. Mangiarotti, J.M.Sierchio P. Bonoli, C. Kasten, D.A. Sutherland, H.S. Barnard, C.B. Haakonsen, J. Goh, C. Sung, D.G. Whyte, “ARC: A Compact, High-Field, Fusion Nuclear Science Facility And Demonstration Power Plant With Demountable Magnets,”Fusion Engineering and Design, 100, November 2015, Pages 378-405
- Click here to go to Technical References for Steven B. Krivit’s JET and ITER Power Investigation.
- EU DEMO Q-values based on G. Federicia, C. Bachmann, L. Barucca, W. Biel, L. Boccaccini, R. Brown, C. Bustreo, S. Ciattaglia, F. Cismondi, M. Coleman, Loving, F. Maviglia, B. Meszaros, G. Pintsuk, N. Taylor, M.Q. Tran, C. Vorpahl, R. Wenninger, J.H. You, “DEMO Design Activity in Europe: Progress and Updates,” Fusion Engineering and Design, 136 (A) November 2018, Pages 729-741, and communication with H. Zohm.