By Steven B. Krivit
March 26, 2024
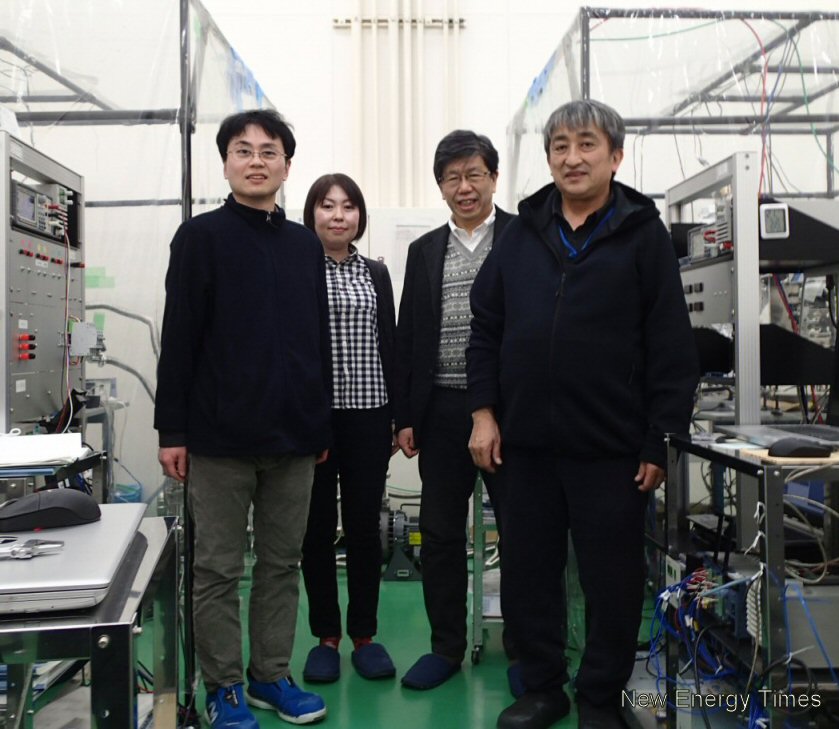
(Left to right) Tohoku University scientists Tomonori Takahashi, Shinobu Yamauchi, Yasuhiro Iwamura, and Takehiko Itoh (Photo: Yuji Tsubori)
In an article published in the Japanese Journal of Applied Physics this month, four researchers at Tohoku University have reported system-wide net energy production in low-energy nuclear reaction (LENR) experiments.
The Tohoku researchers identify the underlying mechanism as an “anomalous heat generation phenomenon.” They do not attribute their results to a nuclear fusion reaction.
Back in 2006, theorists Lewis Larsen and Allan Widom proposed a neutron-based theory to explain LENRs. Thus far, this electroweak-interaction theory has emerged as the most likely explanation for LENRs. The Widom-Larsen theory predicts high reaction rates sufficient to explain the excess heat reported in the field’s 35-year history, beginning with the thermal results published by Martin Fleischmann and Stanley Pons on April 10, 1989. The theory predicts the production of low-mass nuclides such as tritium and helium, heavy-mass nuclides across the periodic table, and shifts in isotopic abundances. It also predicts that the heat production and nuclear products can occur with either normal hydrogen, or heavy hydrogen (deuterium) as the fuel.
The Tohoku benchtop experiment is small and visually unimpressive, but it runs on hydrogen — ordinary, cheap hydrogen, abundantly available on Earth in virtually unlimited quantities. Neither deuterium nor tritium are required. Nor are massive shielding and containment facilities, because no harmful levels of radiation are emitted.
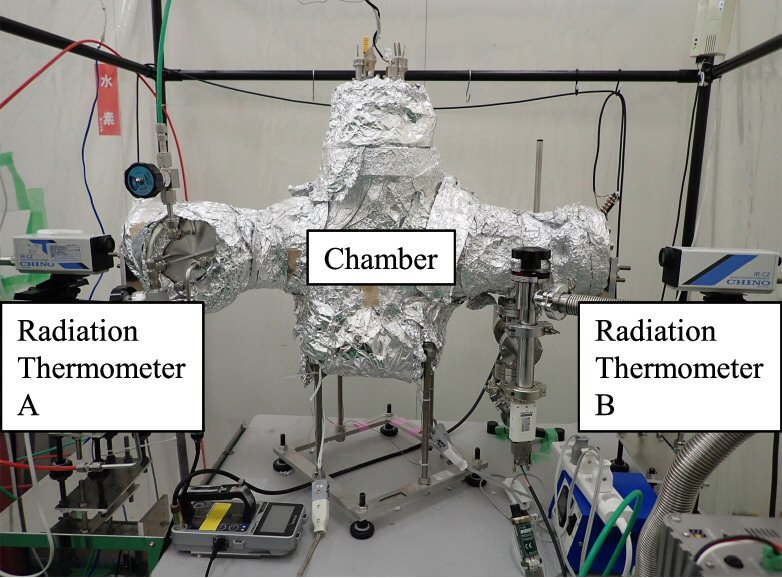
Tohoku University LENR hydrogen-gas experiment, wrapped in insulation
The Tohoku researchers have demonstrated, many times over, thermal output in excess of all electrical heating input. The experiments do not operate in the multi-megawatt range typical of a centralized power plant. Instead, they produce a few Watts of thermal energy that, if developed into electricity producing devices, would be suitable for use in small electronic devices and mobile phones.
Despite their potential, these experiments — at the moment — represent the lowest levels on any technology readiness level scale.
Nevertheless, keeping two historical precedents in mind is useful. The unexplained effect observed by Pierre and Marie Curie a century ago, later found to be the result of nuclear fission, has become one of the world’s major energy sources.
Decades ago, business computing was dominated by mainframe and minicomputers. Along came personal computers, which have now been miniaturized to form multi-thousand-unit clusters in server farms that run some of the largest businesses in the world.
Tohoku LENR Experimental Procedure
The authors of the Japanese research are Yasuhiro Iwamura, Takehiko Itoh, Shinobu Yamauchi, and Tomonori Takahashi. Iwamura and Itoh have been performing LENR research for more than three decades. They worked at Mitsubishi Heavy Industries before moving to Tohoku University. At Mitsubishi, they designed LENR experiments that used deuterium gas permeation that repeatedly produced evidence of nuclear transmutations, a more definitive sign of nuclear reactions than heat production.
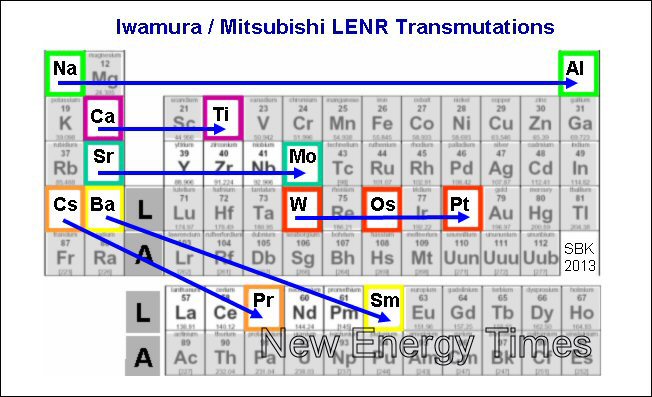
Iwamura and Itoh began their current experiment series in 2016. The heart of the experimental design is a multilayer thin-film metal composite, prepared on a nickel substrate, 100,000 nanometers thick, about the thickness of a sheet of paper. The researchers use a sputtering technique to apply, on top of the substrate, consecutive ultra-thin layers of nickel and copper.
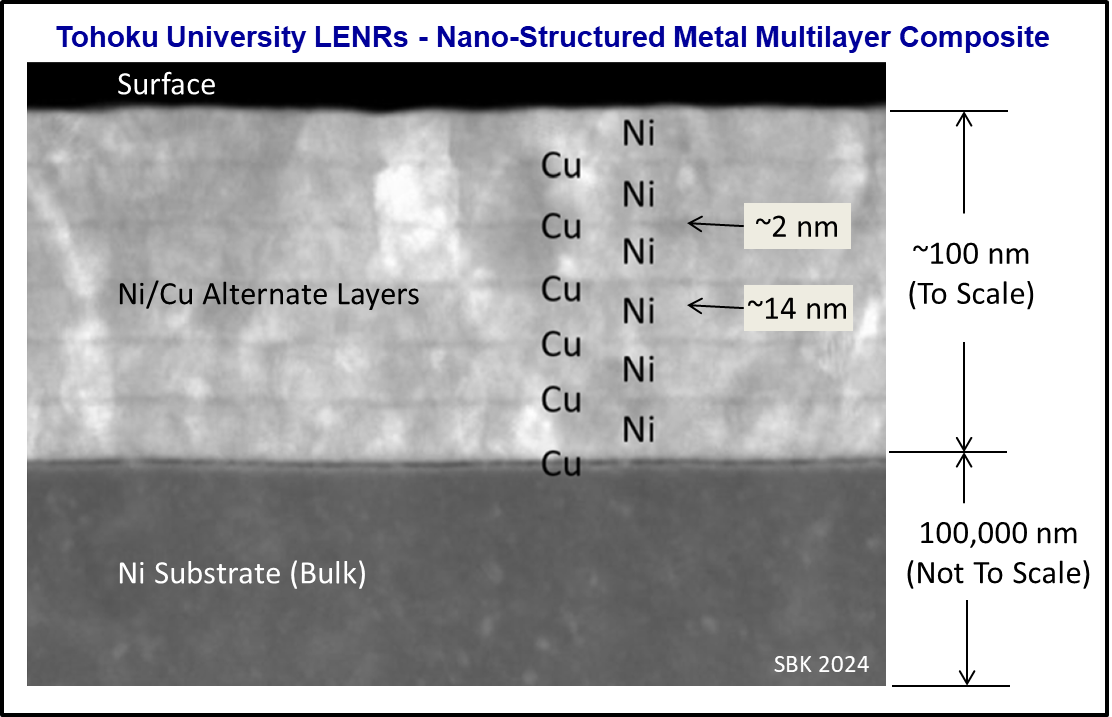
The scientists place this nano-structured metal multi-layered composite inside a reaction chamber and run cyclic experiments. Each cycle has two phases.
In the first phase, the chamber is pressurized with hydrogen gas. During this phase, the sample slowly absorbs hydrogen gas, and, as a result, the pressure in the chamber drops. (See the blue lines in the image below.) The typical absorption time is about 16 hours.
In the second phase, when the hydrogen is fully loaded (absorbed) into the sample, air is evacuated from the chamber. At the same time, heaters are switched on. The input power from the heaters remains constant. During this phase, excess heat (shown by the red lines) is produced. For example, in the first cycle, the input power was 19 Watts, and the excess heat, or net power, was 3.2 Watts and lasted for about 11 hours. At the end of the second phase, the heaters are turned off, and the cycle is restarted.
The procedure differs slightly from experiment to experiment. In the experiment shown below, in the last cycle, the researchers did not turn the heaters off and allowed the second phase to run longer. The excess heat starts at a peak of 3.8 Watts and gradually descends for about 28 hours. This experiment ran for a total of 166 hours. In other papers, the researchers have reported 9 Watts of peak excess heat.
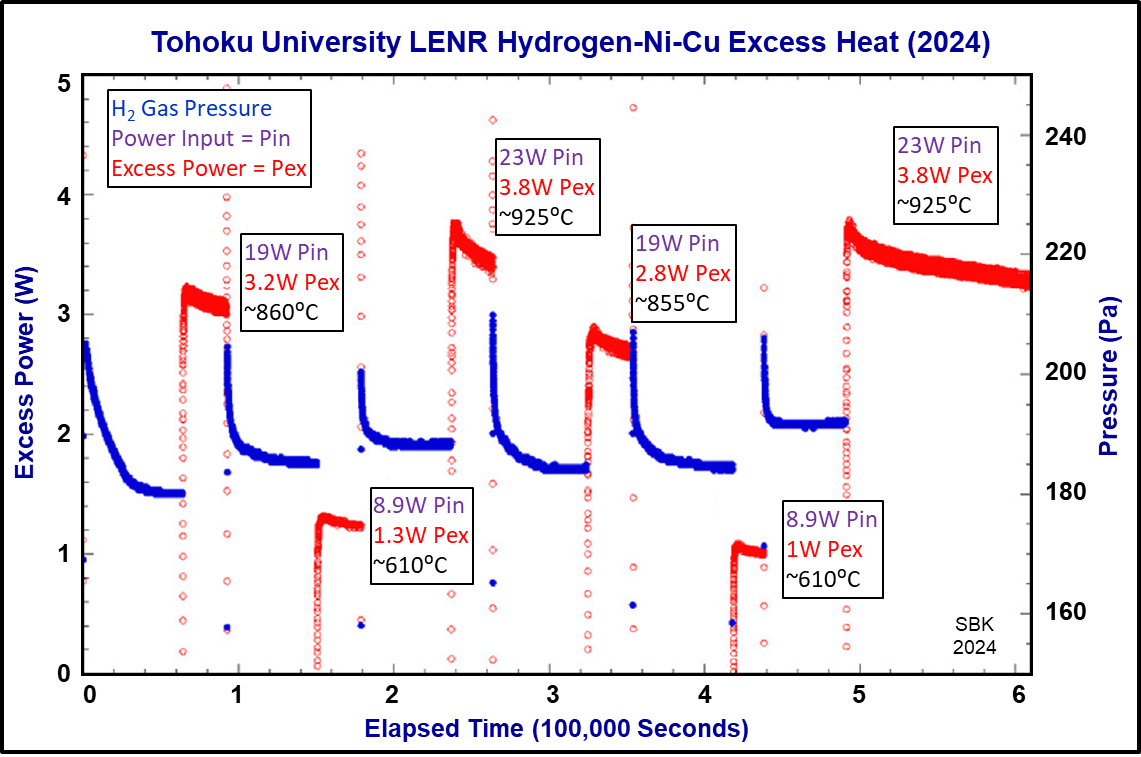
Iwamura told New Energy Times that the integrated value of the input electric power during the entire experiment was 4.8 MJ. The total output of heat generation during the experiment was 5.56 MJ, resulting in a net thermal output of 0.76 MJ.
In previous years, hydrogen or deuterium gas experiments by this and other groups of LENR researchers were designed to produce results during the gas absorption phase rather than the desorption phase. But the Tohoku group, and other LENR researchers who have reported similar results in conference presentations, are seeing better results in the desorption cycle. In conversation with Yasuhiro Iwamura, the lead scientist in the Tohoku group, this writer speculated that better results might be occurring with desorption because loading (absorption) takes much longer than deloading (desorption) and thus a higher hydrogen flux rate is achieved during desorption.
Energy Density
The Tohoku researchers have calculated the energy released in the experiments per gram of hydrogen fuel. In the currently reported experiments, they report a range of 10 to 10,000 megajoules produced per gram of hydrogen. Comparable results were achieved from 2015 to 2017 in a previous collaboration between Kobe University and Technova Inc., a NEDO (New Energy and Industrial Technology Development Organization) project involving researchers from Tohoku University, Nagoya University, Kyushu University, and Nissan Motor Co. The results were published in the International Journal of Hydrogen Energy in 2018. In those experiments, researchers also used the hydrogen (as well as deuterium) gas desorption method. But instead of a multilayer composite, those experiments used nanoparticles. The results were typically in the 1,000 megajoule-per-gram range.
The logarithmic chart below shows that the energy density in these laboratory experiments is currently higher than the most energetic chemical fuel yet below that of nuclear fission and fusion reactions.
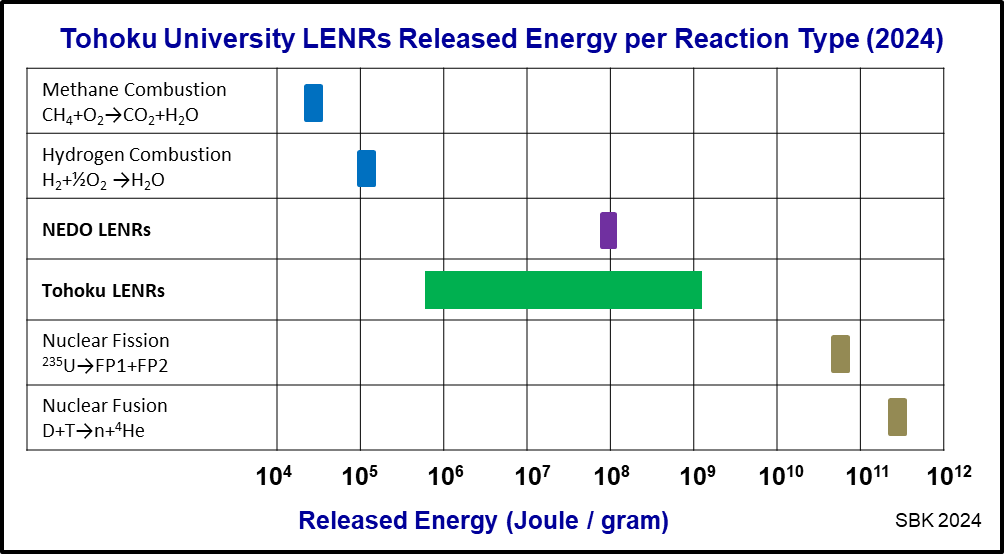
Reproducible
The experiments are not simple or easy. However, Iwamura told New Energy Times that the Tohoku team has repeated the experiment about 200 times. They found that their best configuration is a six-layer composite. With this configuration, Iwamura reports almost 100 percent repeatability. Moreover, when the researchers use a composite that produces significant excess heat, they can repeat the same results with the same composite.
In addition to the previous replications by the NEDO group, Iwamura told New Energy Times that a research and development laboratory operated by one of their financial sponsors, Hideki Yoshino’s Clean Planet company, “has successfully reproduced the experiment with different equipment, different samples, and different researchers.”
Deliberate Trigger
The Tohoku researchers are also beginning to learn how to deliberately trigger small heat bursts by inducing minor variations in the input power. They don’t turn the power up but instead turn it down slightly and momentarily, then return it to the original level.
Iwamura also reports nearly 100 percent repeatability of the deliberate triggering effect. In the graph below, input power was initially steady at 25.8 Watts. The researchers then dropped it to 25.0 Watts, and when they returned it to the original 25.8 Watts, all temperature sensors in the experiment showed a small increase, in the range of 0.8 Watts peak.
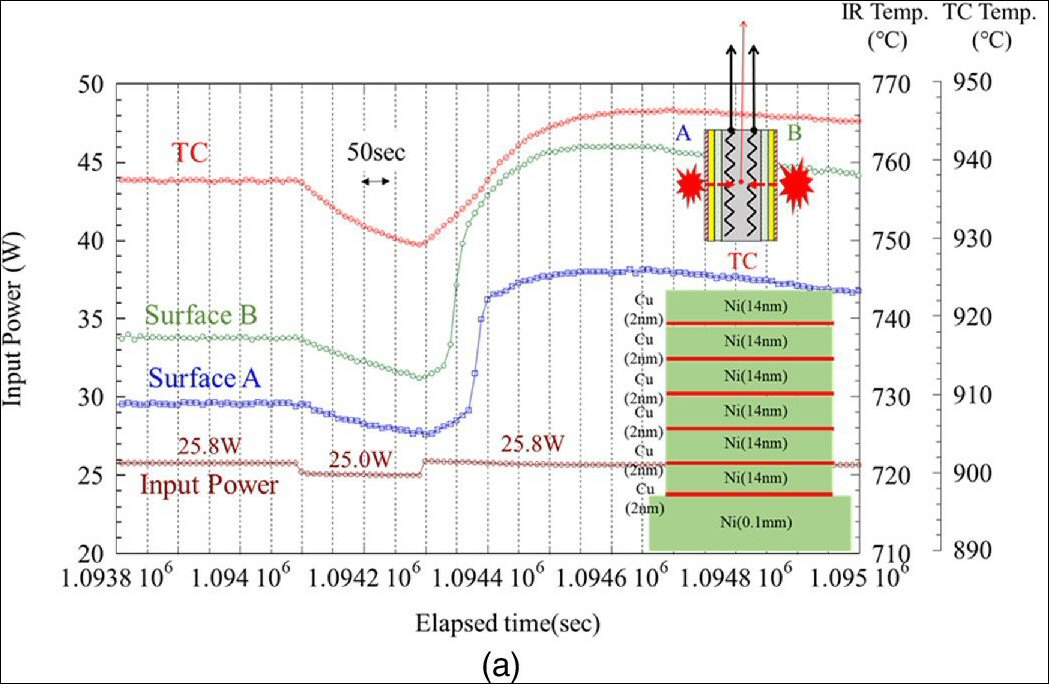
Excess-heat burst in response to intentional power adjustments in Tohoku University experiments
Nuclear Products
The Tohoku group has looked for changes in the abundances of elements and for unusual isotopic ratios after the experiments. Iwamura reports that they have seen many unusual accumulations. However, the most prevalent is an unusually high percentage of the element oxygen showing up below the surface of the multilayer composite, within the outer areas of the bulk.
Pre-experiment analysis for the presence of oxygen concentration, after fabrication of the multilayer composite, has indicated a concentration of 0.5 to a few percent down to 1,000 nm from the top surface. The Tohoku group has observed many accumulations of oxygen in post-experimental analyses exceeding 50% in specific areas.
Iwamura says that once the multilayer is fabricated, there is no way for atmospheric oxygen to leak below the top surface, at least beyond the first few nanometers. As a cross-check, researchers looked for nitrogen (which would suggest contamination from the atmosphere) but they detected no nitrogen in the samples.
The image below shows a cross-section of a sample, rotated 90 degrees, showing the top surface on the left.
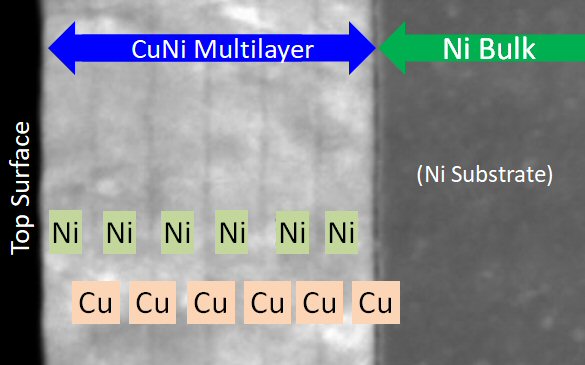
A pair of images from the recent paper, below, show the concentrations of elements, primarily nickel, copper, and oxygen, before and after the experiment.
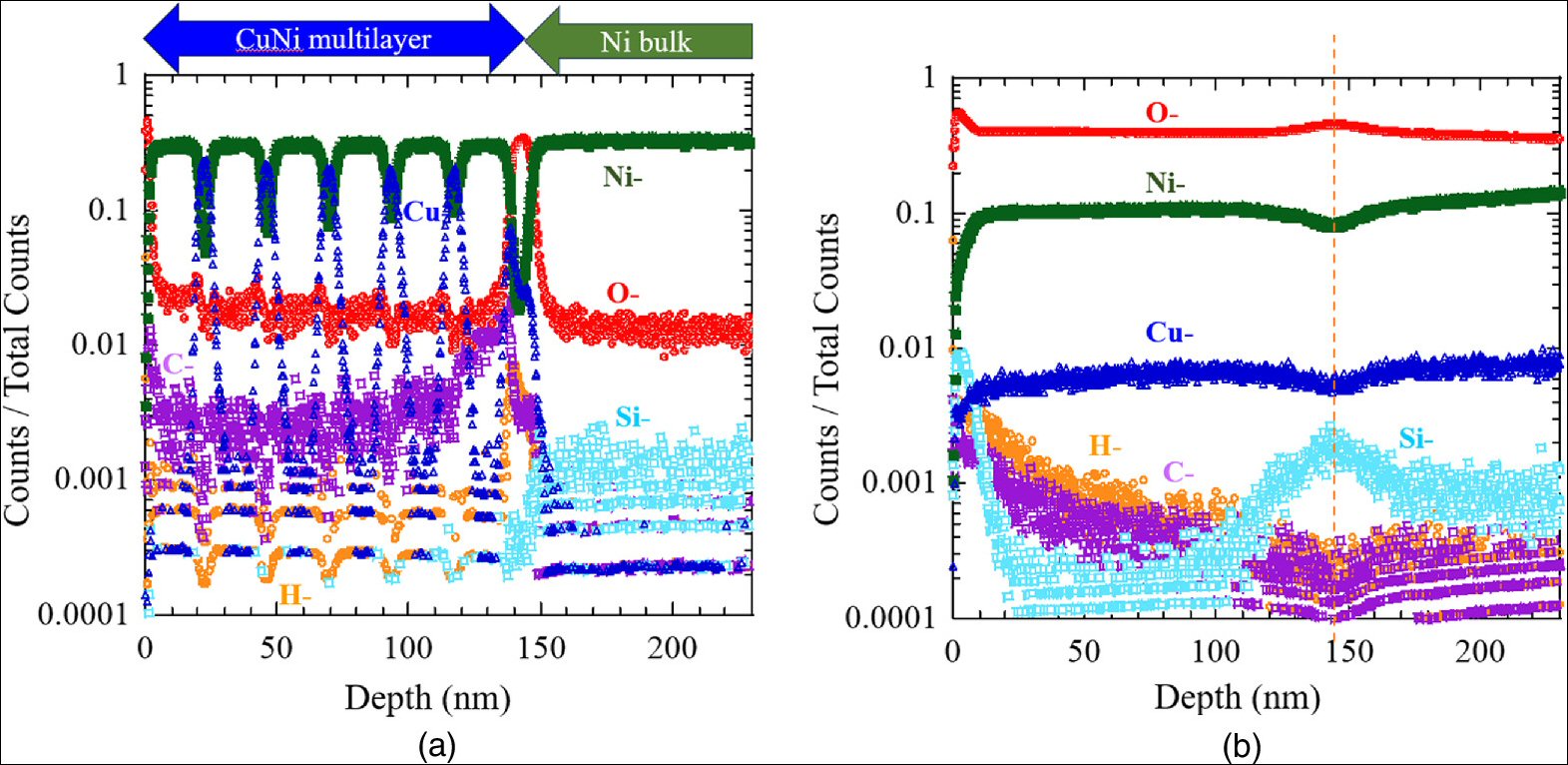
Comparison of depth analysis by TOF-SIMS between samples before and after the experiment; (a) Depth profile of the sample before the experiment and (b) depth profile of the sample after the experiment in which excess heat of up to 9 W was generated. (Text Iwamura et al.)
Before the experiment, (image a) the multiple layers of copper and nickel are clearly visible by the abundances of each element. At the far left, oxygen appears in a narrow peak in the first few nanometers from the top surface. Oxygen also appears at a peak around 145 nanometers from the surface at the interface between the bulk and the multilayer thin films. Otherwise, in this experiment, oxygen generally remains at about the 0.78 percent concentration, relative to the abundances of other elements, on this log scale.
After the experiment, (image b) the concentration of oxygen is nearly flat and is up at the 50 percent level on this log scale. In addition, almost as if the metals had melted, and been drawn across the composite, the peaks of nickel and copper all disappear, and concentration of each element evens out. There is also a significant increase of copper in the bulk, that is, beyond the 150 nanometer depth. New Energy Times asked Iwamura for details about the increase in copper.
“The EDX analysis shows that the percentage was less than 1 percent before the experiment, but several tens of percent after the experiment. Several EDX results are documented in the paper,” Iwamura said.
Science Marches On
Iwamura and his colleagues are among hundreds of LENR researchers who have remained steadfast in the past 35 years despite intense hostility, mockery, ridicule, and extraordinary constraints on funding and publishing. The conflict is understandable. In 100 years of chemistry and physics, most scientists have thought nuclear reactions could occur only in high-energy physics experiments and in massive nuclear reactors. But LENR research shows otherwise: Nuclear reactions can also occur in small, benchtop experiments. This is contrary to the beliefs and experience of physicists and chemists, although not contrary to the science and experimental findings.
Through the efforts of these researchers and of many first-generation LENR researchers who are no longer alive, we have the opportunity today to witness the development of a new field of science, a new field of nuclear research, and a possible solution to energy scarcity and climate change.
New Energy Times asked Iwamura what he would like to achieve next.
“I would like to elucidate the mechanism of what is happening while at the same time work on the development necessary for practical application,” Iwamura said.
Iwamura will be presenting his team’s findings at the LENR session at the American Nuclear Society national meeting in Las Vegas in June.
Want To Learn More?
“I do not remember any case in my lifetime in science when so many experts have differed for such a long time on such relatively simple and inexpensive experiments.” — Edward Teller. Oct. 16, 1989
Edward Teller (1908-2003), the father of the hydrogen bomb, said those profound words only six months after the announcement of the excess-heat discovery by Martin Fleischmann and Stanley Pons, igniting the biggest controversy in modern science.
Readers who are interested in the most accurate and complete accounts of this history and science will enjoy Steven B. Krivit’s Explorations in Nuclear Research three-book series (Hacking the Atom, Fusion Fiasco, Lost History) Collectively, they describe the emergence of a new field of science, one that bridges chemistry and physics.
The books give readers an understanding of low-energy nuclear reaction (LENR) research and its history and provide a rare behind-the-scenes look at the players and personalities involved. The books present the results of in-depth historical research and draw on formerly inaccessible archives to describe what occurred in the research that has been mistakenly called “cold fusion.”
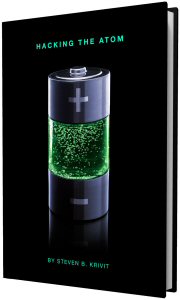
Hacking the Atom: Explorations in Nuclear Research, Vol. 1
Making sense of the new science of low-energy nuclear reactions (1990-2015)
Hacking the Atom, written for scientists and non-scientists alike, covers the period from 1990 to 2015 and explains how changes to atomic nuclei can occur with low-energy methods. The book reveals the hidden story of how the science initially and erroneously called “cold fusion” continued to progress slowly but incrementally after its near-death in 1989. The book shows that 100 years of chemistry and physics is not wrong but is incomplete and that there is something new and exciting in the physical sciences.
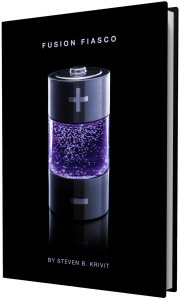
Fusion Fiasco: Explorations in Nuclear Research, Vol. 2
The definitive historical account of the “cold fusion” conflict (1989-1990)
Fusion Fiasco, written for scientists and non-scientists alike, covers the period from 1989 to 1990, and tells the most accurate and complete story of the 1989 to 1990 “cold fusion” conflict. Relying heavily on archival records, the book documents one of the most divisive scientific controversies in recent history. The book explains why credible experimental LENR research emerged from the erroneous idea of room-temperature fusion, as claimed by Martin Fleischmann and Stanley Pons at the University of Utah.
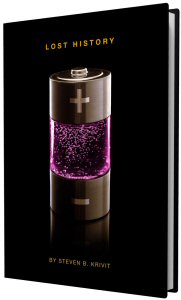
Lost History: Explorations in Nuclear Research, Vol. 3
The forgotten story of chemically induced nuclear transmutations (1912-1927)
Lost History, written for scientists and science historians, covers the period from 1912 to 1927, and explores the story of forgotten transmutation research, a precursor to modern low-energy nuclear reactions (LENR) research. The book tells the story of century-old research that has been absent from the scientific dialogue for a hundred years — research that is surprisingly similar to events in the modern era.