EPRI-Ahern Report: LENR Is 45 Times More Energetic Than Diesel
Sept. 27, 2012 – By Steven B. Krivit –
[This is the fourth of a four-part series. Part 1 is here. Part 2 is here. Part 3 is here.]
This article reports on low-energy nuclear reaction research performed by Brian Ahern, an independent researcher from Boxborough, Mass. Ahern has been researching LENRs for the past four years. He has worked on the nanomaterial and hydrogen-isotope-gas-absorption LENR method. His LENR research was partially sponsored by the Electric Power Research Institute, an independent research company that provides technology, policy and economic analyses to the companies that produce 90 percent of the electricity generated and delivered in the United States.
Ahern’s intention was to replicate the nanomaterial and hydrogen-isotope-gas-absorption experiment developed by professors Yoshiaki Arata and Yue-Chang Zhang at Osaka University beginning in 2005. (See New Energy Times story here.)
Since then, other groups, mostly in Japan, attempted replications of the Arata/Zhang work. These included a Technova-sponsored group at Kobe University, as well as a group at the Toyota Central Research and Development Laboratories in Nagakute, Aichi, Japan. (See the second and third articles in this four-part series.)
When it comes to LENR nanomaterials, Ahern has the right stuff. He earned his master’s degree in physics at the University of Vermont. His thesis was “Superconduction in the Palladium Hydride-Palladium Deuteride System.” He has a Ph.D. from the Massachusetts Institute of Technology in materials science. He has several related U.S. patents, and he performed electromagnetic materials research for the U.S. Air Force in its Rome laboratory for 18 years. Ahern is an independent and creative thinker and has brought fresh insights to LENR research.
LENR caught his attention in 2008 after he heard about the Arata/Zhang LENR work. Because of his history with nanopowders, he recognized something familiar, as he explained to New Energy Times.
“Back in 1996, my colleagues and I at MIT made a discovery, which we later patented, with a nanoparticle exchange mechanism. In the process, we figured out that something was very important about the 3- to 12-nanometer size range. So when I read that Arata was using 5- to 10-nanometer particles, I knew he was right in the middle of that sweet spot, and I decided to make material in that same scale and try to find out if he was right,” Ahern said.
2008 – Ahern and Takahashi Discuss Nanomaterials
Ahern did not attend ICCF-14. He first read the Arata/Zhang paper in November 2008. He also learned about another ICCF-14 paper in which a group at Kobe University in Japan performed an Arata/Zhang replication attempt.[1]
“We have constructed an experimental system to replicate the phenomenon of heat and 4He generation by D2 gas absorption in nano-sized Pd powders reported by Arata, and to investigate the underlying physics,” the authors wrote.
However, their results were ambiguous and didn’t give them confidence.
“When D2 gas was used with Pd-black, apparent excess-heat production was observed. However, temperature oscillations and drift were relatively large, so the accuracy of the system must be improved to confirm the result,” they wrote.
Ahern told New Energy Times that he recognized the problem right away: The Kobe group had used 100-nm-sized particles. He contacted Akito Takahashi, professor emeritus at Osaka University, who had joined Technova as a senior adviser in 2007. Takahashi, through Technova, helped fund that LENR research at Kobe University through a project he called Metal Deuterium Energy. Takahashi is well-known in the LENR field for his proposed theory of deuterium-deuterium “cold fusion.”
Ahern sent an e-mail to Takahashi on Nov. 10, 2008.
“I have studied your recent article replicating Arata’s work,” Ahern wrote. “I ask you to please repeat the experiment with the proper palladium nanopowders. I am an expert on nanotechnology, and I predicted that the nanopowders must be in the 5-8 nm range in order to maximize the effect. Your powders were much too large so they acted like bulk palladium.”
Takahashi replied to Ahern the next day.
“Thank you for your advice,” Takahashi wrote. “We are very well aware of what you suggested. However, Arata is right now rejecting using his nano-particle powders for our experiment. So we need to prepare similar ones from somewhere else. This is taking time. Anyway, we will proceed with our work to test nano-sized samples.”
Takahashi did not explicitly tell Ahern that he knew the importance of the 4-10 nanometer particle size, so Ahern continued to offer his advice and encouragement. He told Takahashi that he had been the U.S. Air Force’s resident expert on nanotechnology, and he offered his expertise to Takahashi. In a Nov. 11, 2008, e-mail, Ahern told Takahashi where he could purchase the right size materials. Ahern also sent Takahashi a paper by “Lahiri”, (he did not remember the researcher’s full name), that Ahern said supports the role for nanopowders in the correct size regime.
Takahashi thanked Ahern for the Lahiri paper and told Ahern that he would read it to study and learn.
Ahern sent another e-mail to Takahashi and discussed ideas developed by Keith H. Johnson, emeritus professor of materials science from MIT. Ahern sent a summary of a 1993 paper by Johnson and commented on one of Takahashi’s related theoretical assumptions.
“That is the essence of the problem,” Ahern wrote. “The [Born-Oppenheimer] approximation is invalid for the deuterons in palladium. Professor Keith H. Johnson described this in his 1983 treatise on superconductivity. Everything points to nanopalladium as being the ideal host for supporting nuclear interactions. The oscillations of the hydrogen isotopes are optimized.”
Ahern continued sending information to Takahashi. On Nov. 15, 2008, Ahern listed six technical reasons why 4- to 10-nanometer-size particles were important.
“In conclusion,” Ahern wrote, “processing palladium into a narrow-size distribution in the 4- to 10-nm size regime offers the best opportunity for developing clean and limitless nuclear energy.”
[Ahern’s photograph removed in response to DMCA request from EPRI]
Nanopowders Used by Ahern in EPRI-Sponsored LENR Experiments
Ahern sent other technical details, slides and some technical animations to Takahashi. Their e-mail exchange continued until at least Jan. 8, 2009.
“I hope I have given you enough information to inspire a replication of the Arata experiment with powders in the proper size regime,” Ahern wrote. “If you need assistance in finding a source, there is a commercial source in the Chicago area. … Have you tried to use powders in this dimension yet? I believe that anything over 20 nm will act like bulk materials.”
Takahashi did not answer Ahern’s question about particle size. Their e-mail exchange also shows that they both had difficulty obtaining the same material that Arata had used.
“I want to replicate the Arata experiment as exactly as possible,” Ahern wrote. “I have e-mailed professors Arata and Zhang seeking to purchase some powders (5 nm Pd in ZrO2), but they have not responded.”
Takahashi had similar difficulties.
“That company in Kyoto (Fukuda Fine Metal Powder Co.) is not allowed to provide the sample to us, as Arata refuses to provide it,” Takahashi wrote. “We have obtained similarly fabricated samples from a company in Kobe (we cannot tell the name yet) and are doing experiments.”
On March 23, 2009, at the American Chemical Society meeting in Salt Lake City, Utah, Takahashi reported his group’s successful replication.[2]
2009 – EPRI Sponsors Ahern
One month after the ACS meeting, EPRI agreed to sponsor Ahern’s attempt to replicate the Arata/Zhang experiment.[3]
In the basic Arata/Zhang design, the experiment has no electrical power input, and once the hydrogen isotope gas is loaded into the metal nanopowders inside the chamber, there is no gas flow. Ahern’s cell consists of a thick-walled Dewar that contains the nanomaterials. Ahern later modified the experiment and heated it to perform experiments above room temperature.
[Ahern’s photograph removed in response to DMCA request from EPRI]
Dewar chamber that houses the nanopowder. Heating tape is wrapped around the outside.
The key to working with nanopowders in these experiments is to keep them from agglomerating, particularly when the experiments get hot. If the particles clump, they stop working – that is, the experiment doesn’t produce anomalous heat. Researchers have known for a long time that high surface areas on the material used in LENRs are essential and that, without them, anomalous heat usually does not occur. Ahern said that materials with a particle size larger than 20 nanometers act like bulk materials and take a long time to show excess heat, if ever.
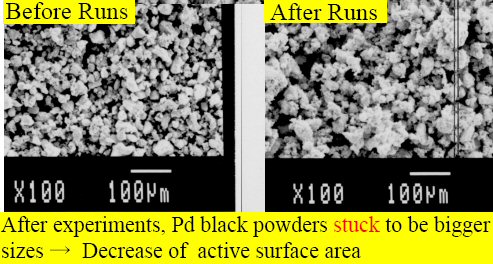
Agglomeration of nanopowders. Image courtesy Akito Takahashi, presented at American Chemical Society, March 2009
Ahern used two forms of nickel-alloy nanomaterials (using a variety of alloy compositions) in this series of experiments. The first was a nickel-alloy nanocomposite made of nickel, other metals and zirconium oxide. The second nanomaterial was a nickel-alloy nanopowder from Quantum Sphere Corp.
Ahern explained the nanocomposite using an analogy.
“The nanocomposite is like raisin bread, where the raisins are equivalent to the nickel-alloy islands and the zirconium oxide is equivalent to the bread,” he said. “The bread keeps the raisins from touching each other. In the alloy, if the particles end up touching each other, they coalesce, so you need some isolation material, and the zirconium oxide does that.”
[Ahern’s photograph removed in response to DMCA request from EPRI]
Transmission electron microscopy photomicrograph of Ahern’s nanocomposite material.
Hydrogen Isotopes
Ahern had been under the impression that Arata’s best results had occurred with the nickel-hydrogen system.
The Ahern-EPRI paper says, “Arata’s work with nickel and hydrogen had many similarities to work reported by Piantelli and Focardi in 1994.”
However, unlike Piantelli and Focardi, Arata had never made a claim of excess heat using the nickel-hydrogen system. A claim of excess heat with hydrogen rather than deuterium would negate Arata’s claims of his “cold fusion” discovery. In a 2008 paper with Zhang, Arata asserts a priority with “cold fusion,” which he calls “solid fusion,” that pre-dates Martin Fleischmann and Stanley Pons’ 1989 announcement.
“About 50 years ago (1955~1958), one of the authors (Y. Arata) investigated ‘solid-state plasma fusion’ (simply ‘Solid Fusion’) together with ‘thermonuclear fusion’ (simply ‘hot fusion’). He was the first researcher in the world to discover ‘Solid Fusion,’ as well as the first researcher in Japan to discover ‘Hot Fusion,'” the authors wrote.
After a discussion with this writer, Ahern agreed that he had been mistaken about his perception of Arata’s claim. Somehow, none of the reviewers of the EPRI paper caught this error.
Ahern explained to New Energy Times that he switched his attempts to get heat from the palladium-deuterium system to the nickel-hydrogen system not only as a result of his impression that Arata had made a similar claim but also for practical reasons.
“I gave up on deuterium early on,” Ahern said. “If it works with hydrogen, why bother with deuterium? Why use palladium if it works with nickel?”
Ahern had also known that Piantelli had done his work exclusively with the nickel-hydrogen system and obtained great results with those materials.
Ahern’s unintended variation on Arata’s experiment to seek excess heat from the nickel-hydrogen system turned out to work strongly in his favor. Not only did he get his highest levels of excess heat with the nickel-hydrogen system, but he also sent his nickel nanocomposite material to Kobe University in Japan for confirmation. The Kobe researchers used Ahern’s material in a LENR gas-absorption experiment, and they too got strong results. As a side benefit, they provided a mostly independent confirmation of Ahern’s experiment.
However, excess heat with hydrogen rather than deuterium does not support Takahashi’s theory of deuterium- deuterium “cold fusion.” This poses a significant conflict for Takahashi, who, through Technova, sponsored the Kobe work.
Thermal Triggering
Ahern explained his experimental process to New Energy Times. He fills the experimental chamber with deuterium or hydrogen gas to 200 psi, then waits until the pressure drops. This indicates that the gas has absorbed into the metal. Then he goes through more filling cycles, sometimes up to 10 before the nanomaterial fully loads. This process may take place over half an hour. Initially, he ran his experiments at room temperature, as he explained to New Energy Times.
“For the first two years, I was just looking at what happens when you add hydrogen or deuterium,” Ahern said. “You watch it heat up as it loads in and makes an exothermic compound, and then it is supposed to cool back to room temperature. The thing is that it doesn’t cool back to room temperature. It stays about half a degree to 2 degrees above room temperature, apparently indefinitely.
“But that’s all the energy you get at room temperature. It’s equivalent to 100 to 200 mW. But once it goes above 360 degrees C, you start to see it produce 5 to 8 Watts of excess heat. Keep in mind that it needs 50 Watts to get it to that temperature, so the percent of excess heat is not very exciting. This has no practical use, but it is good for scientific research.”
Ahern said his experiments were easily repeatable.
“My replications of the Arata experiments at room temperature were 100 percent repeatable,” he said. “I could run them 14-15 times in row, but they were very unspectacular. There was no reason to do more of that style of experiment. It was repeatable — and negligible.”
New Energy Times asked Ahern whether he had data or tables for his elevated-temperature experiments because that work does not appear in his EPRI report. Unfortunately, he did not have a copy of that data set because of a computer failure.
Toward the end of Ahern’s series of experiments, he heard from some Italian experimenters about the possible use of a copper additive to nickel-hydrogen LENR experiments.
“So I went and looked up copper, and I discovered a paper from Romanowski from 1999 where he said that, if you want to get the most hydrogen broken up, you really want to use copper and nickel,” Ahern said. “The reason is that hydrogen is a diatomic molecule. It wants to stay diatomic, but it has a very low solubility. If you break it into atomic hydrogen — individual hydrogen atoms — then it can dissolve into the material. A copper-nickel alloy does this best.”
Ahern obtained good results with the copper additive and again sent his new material to Kobe University so the researchers there could attempt to replicate the result, and they did.
In Ahern’s final experiment reported in the EPRI paper, he switched from using the nanocomposite to Quantum Sphere Corp.’s nanopowder spheres. He gave another food analogy: He said the spheres are like M&M chocolate candies. They spheres are dense arrays of stacked particles, but they keep from melting together during an experiment because of the thin oxide coating covering each one.
Ahern obtained a spectacular result with this nickel nanomaterial and hydrogen gas. Even though he later lost the computer data, he knew at the time that he was seeing an important event, and he made a screen capture.
[Ahern’s photograph removed in response to DMCA request from EPRI]
Screen capture of Ahern experiment with nickel alloy nanopowder that produced 21 watts of excess heat from 5 grams of nanopowder and hydrogen gas for 5 days.
In his configuration, the temperature sensor on the outside, which is in contact with the heating tape, normally reads 20 degrees C hotter than the inner temperature sensor. At one point during the experiment, Ahern saw the inside temperature jump to 280 degrees C while the outer sensor stayed at 250 degrees C. A few hours later, both sensors simultaneously showed a rapid rise of heat. The outer sensor hit a plateau of 325 degrees C. The inner sensor went up to 450 degrees C, hit a plateau for a few minutes, then jumped again, this time up to 533 degrees C.
Ahern wrote in the report that the run maintained that level of heat output for five days until he intentionally terminated it. He told New Energy Times that he ran 50 Watts of electrical heat into the system and measured 71 Watts heat output. He stated that it produced roughly 21 watts of heat from 5 grams of nanopowder. He calculated the energy release (21 Watts x 5 days x 86,400 seconds) at 9 Mj from the 5 grams of material and hydrogen.
“The most energetic substance on earth is diesel fuel, and it gives off 40 kJ per gram,” Ahern said. “So anything over 200 kj for the 5 grams of material I used would be beyond any known chemical reaction. In this case, it produced 45 times more energy per gram of material than diesel fuel.”
1. T. Nohmi, Y. Sasaki, T. Yamaguchi, A. Taniike, A. Kitamura, A. Takahashi, R. Seto, Y. Fujita, “Basic Research on Condensed Matter Nuclear Reaction Using Pd Powders Charged With High Density Deuterium,” ICCF-14, 2008
2. Kitamura, Akira, Nohmi, Takayoshi, Sasaki, Yamaguchi, Tatsuya, Yuuki, Taniike, Yuuki, Taniike, Akira, Takahashi, Akito, Seto, Reiko, Fujita, Yushi, “Deuterium Gas Charging Experiments with Pd Powders for Excess Heat Evolution,” ACS 2009
3. Rastler, Dan and Ahern, Brian, “Program on Technology Innovation: Assessment of Novel Energy Production Mechanisms in a Nanoscale Metal Lattice,” EPRI Technical Update, 1025575, August 2012
Questions? Comments? Submit a Letter to the Editor.